Aging is a multifactorial and complex process; indeed, it is probably the most complex disorder known. It is made up of a combination of dozens of malfunctions locked together in an accelerating spiral of decline. Despite this undeniable complexity, which is captured to some extent by grouping the many age-related dysfunctions into nine categories often termed the “hallmarks of aging”, aspects of age-related molecular and cellular decline are often described and approached as distinct mechanisms [1].
Distinct mechanisms are difficult to reconcile with the substantial biological complexity of aging. Each “hallmark of aging” is comprised of processes that are undertaken by many networks of interacting proteins, and each network itself involves hundreds of protein species. Even at the organismal level, the Hallmarks are clearly not discrete entities but are themselves interconnected and overlapping. Trying to pick out single mechanisms embedded in the enormous cascade of complexity that underlies age-related decline might not be the most profitable approach imaginable.
This has been exemplified recently by the identification of links between three major manifestations of aging that have often been studied separately: NAD+ decline, cellular senescence, and chronic inflammation [2].
NAD+, cellular senescence and chronic inflammation
NAD+ is a ubiquitous molecule that plays a key role in multiple biological processes, ranging from energy metabolism to cell survival and repair. It is now known that cellular NAD+ levels decline with age and that this decline plays a direct role in the development of age-related metabolic dysfunction and disease [3]. Meanwhile, cellular senescence is a tumor-suppressive response that has evolved to prevent the unrestricted division of damaged and potentially cancerous cells.
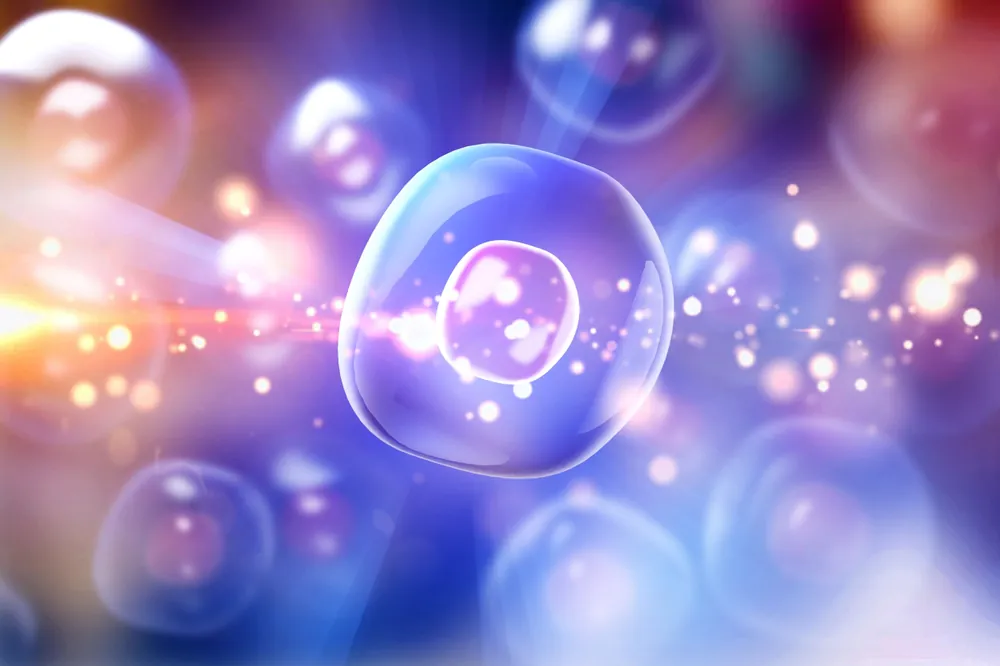
Read More
Both restoration of NAD+ levels and the selective elimination of senescent cells independently promote health and extend lifespan in old or diseased animals [4, 5, 6]. Strategies to develop therapies to individually mitigate each of these hallmarks of aging are being developed.
Recent studies, however, have demonstrated that falling NAD+ levels and senescent cell recruitment are linked. This link appears to be mediated via another key hallmark of aging: chronic inflammation [1, 7].
Crosstalk between Hallmarks
It has recently been demonstrated that high NAD+ in cultured senescent cells increases their secretion of pro-inflammatory SASP cytokines due to inhibition of AMPK by high NAD+. This inhibition leads to downregulation of p53, activation of p38 MAPK, and upregulation of pro-inflammatory NF-kB [1].
This link between NAD+, senescence and inflammation deserves careful consideration. This observation is not evidence that high NAD+ causes senescence: in fact, NAD+ levels and senescence are strongly negatively correlated through life [8, 9, 10]. It is, however, evidence that NAD+ has a role in the biology of senescent cells, just as it has a role in all cells. Keeping in mind the complex, cascading nature of aging, is it also the case that senescence and the inflammatory SASP are drivers of age-related cellular NAD+ decline?
CD38 and chronic inflammation
Inflammatory SASP factors lead to increased expression of the cell membrane protein CD38 [7]. CD38 is found throughout the body with high expression on the surface of immune cells [11]. Its expression is robustly induced during immune cell activation, playing an important role in multiple aspects of the inflammatory response, including cell migration, activation, antigen presentation and cytokine release [12].
To perform its functions, CD38 consumes NAD+ in great quantities [13]. The accumulation of senescent cells with age leads to increased pro-inflammatory SASP. Increased SASP leads to spiraling levels of CD38 expression and a concurrent decrease in NAD+ levels [7].
Consistent with this effect of senescence on NAD+ levels, the expression and activity of CD38 increases during aging, paralleling the decrease in NAD+ levels [13, 14]. Aged wild-type mice have also been shown to have about half the NAD+ levels of young mice, whereas CD38 knockout mice maintain their NAD+ levels and are resistant to the negative effects of a high fat diet, including liver steatosis and glucose intolerance [15, 16]. Conversely, mice over-expressing CD38 have lower levels of NAD+, defective mitochondria, decreased oxygen consumption, and increased lactate production [14, 15].
The profound effect of CD38 on NAD+ levels is due to CD38 being an inefficient cyclase: CD38 must degrade nearly one hundred molecules of NAD+ to generate just one molecule of cADPR. CD38 is recognised as the primary NAD+ consumer in mammalian tissues [17].
Ultimately, this means that strategies to even slightly inhibit CD38 may lead to substantial increases in cellular NAD+ levels. In support of this, when CD38 is inhibited by flavonoids (e.g. luteolinidin, kuromanin, luteolin, quercetin and apigenin; IC50 <10 μM), a 50% increase in NAD+ is observed [16, 18]. Apigenin increases NAD+ levels in multiple tissues, decreasing global proteome acetylation and improving glucose and lipid homeostasis in obese mice, by increasing the activity of SIRT1 and SIRT3 [14, 16]. The CD38 inhibitor 78c has also been found to reverse age-related NAD+ decline and improve several physiological and metabolic parameters of aging, including glucose tolerance, muscle function, exercise capacity, and cardiac function in mouse models of natural and accelerated aging [19].
Resolving the NAD+ pro-inflammatory paradox
It is evident, therefore, that the relationship between these hallmarks of aging is complex. On one hand, NAD+ appears to facilitate the pro-inflammatory phenotype of senescent cells, while on the other hand, the pro-inflammatory SASP rather directly contributes to the demise of NAD+. The relationship between the two appears to be intertwined via chronic inflammation and CD38.
A key point is that the body is always in a dynamic equilibrium between different processes. Levels of biological molecules are not always good or always bad, they are beneficial or harmful depending on the current state of that equilibrium.
This is exemplified by senescent cells themselves. At first, senescent cells are beneficial to the body, as senescence lowers the risk of cancer. However, if these cells are not cleared away by the immune system, they begin to accumulate and the equilibrium shifts towards tissue damage, promoting inflammation and the further recruitment of senescent cells.
Similarly, in most circumstances, high NAD+ is beneficial, as demonstrated by a large number of studies that show that it promotes higher DNA repair, thus reducing DNA damage and senescence and generally robustifying cells, as is the case in youthful organisms [20, 21, 22, 23]. However, if the older body has already developed senescent cells, high NAD+ might reinforce their ability to secrete inflammatory factors.
With this in mind, some circumstances can be studied in which higher NAD+ might contribute to senescence-induced inflammation. However, the balance of risk is certain to lie the other way: if high NAD+ caused senescence-induced inflammation, then inflammation would reduce with age, as NAD+ does, but the reverse is true [24].
Other circumstances can be studied in which CD38 is suppressed while NAD+ levels are raised. In this circumstance, even if senescent cells are present in older tissues, the multiple benefits of restored NAD+, in increased DNA repair and cellular maintenance functions, for example, can be gained without powering up inflammatory processes [14, 16, 19].
Viewed at low resolution, it is possible to see parallels between the “generally good, but possibly sometimes less so” quality of restored NAD+ levels with the generality of oxygenated blood and nutrients being very beneficial – except when cancer is present, highlighting the danger of dissecting biological systems into their constituent parts without considering the wider context.
Conclusion
It is now evident that the hallmarks of aging are not discrete entities but are instead interconnected and interdependent networks. In light of this, strategies that try to address aging by focusing on isolated proteins, pathways or hallmarks are clearly not going to be enough. Even if we imagine that one process could be mitigated completely (100% amelioration of the accumulation of senescent cells, for example), all the other elements of the cascade would proceed to their unfortunate conclusion.
If we’re going to stop or reverse aging, we’re going to have to look at the deep-rooted causes of aging and understand the complex interplay between them. If the goal is to affect aging beneficially, tackling only one thing will almost always be insufficiently effective. Instead, several factors must be addressed at once, targeting not just one hallmark but simultaneously targeting a substantial proportion of the cascading network of age-related decline.
Literature
[1] López-Otín, C., Blasco, M. A., Partridge, L., Serrano, M., & Kroemer, G. (2013). The hallmarks of aging. Cell, 153(6), 1194–1217. https://doi.org/10.1016/j.cell.2013.05.039
[2] Nacarelli, T., Lau, L., Fukumoto, T., Zundell, J., Fatkhutdinov, N., Wu, S., Aird, K. M., Iwasaki, O., Kossenkov, A. V., Schultz, D., Noma, K. I., Baur, J. A., Schug, Z., Tang, H. Y., Speicher, D. W., David, G., & Zhang, R. (2019). NAD+ metabolism governs the proinflammatory senescence-associated secretome. Nature cell biology, 21(3), 397–407. https://doi.org/10.1038/s41556-019-0287-4
[3] Rajman, L., Chwalek, K., & Sinclair, D. A. (2018). Therapeutic Potential of NAD-Boosting Molecules: The In Vivo Evidence. Cell metabolism, 27(3), 529–547. https://doi.org/10.1016/j.cmet.2018.02.011
[4] Zhang, H., Ryu, D., Wu, Y., Gariani, K., Wang, X., Luan, P., D’Amico, D., Ropelle, E. R., Lutolf, M. P., Aebersold, R., Schoonjans, K., Menzies, K. J., & Auwerx, J. (2016). NAD⁺ repletion improves mitochondrial and stem cell function and enhances life span in mice. Science (New York, N.Y.), 352(6292), 1436–1443. https://doi.org/10.1126/science.aaf2693
[5] Mills, K. F., Yoshida, S., Stein, L. R., Grozio, A., Kubota, S., Sasaki, Y., Redpath, P., Migaud, M. E., Apte, R. S., Uchida, K., Yoshino, J., & Imai, S. I. (2016). Long-Term Administration of Nicotinamide Mononucleotide Mitigates Age-Associated Physiological Decline in Mice. Cell metabolism, 24(6), 795–806. https://doi.org/10.1016/j.cmet.2016.09.013
[6] van Deursen J. M. (2019). Senolytic therapies for healthy longevity. Science (New York, N.Y.), 364(6441), 636–637. https://doi.org/10.1126/science.aaw1299
[7] Chini, C., Hogan, K. A., Warner, G. M., Tarragó, M. G., Peclat, T. R., Tchkonia, T., Kirkland, J. L., & Chini, E. (2019). The NADase CD38 is induced by factors secreted from senescent cells providing a potential link between senescence and age-related cellular NAD+ decline. Biochemical and biophysical research communications, 513(2), 486–493. https://doi.org/10.1016/j.bbrc.2019.03.199
[8] van der Veer, E., Ho, C., O’Neil, C., Barbosa, N., Scott, R., Cregan, S. P., & Pickering, J. G. (2007). Extension of human cell lifespan by nicotinamide phosphoribosyltransferase. The Journal of biological chemistry, 282(15), 10841–10845. https://doi.org/10.1074/jbc.C700018200
[9] Borradaile, N. M., & Pickering, J. G. (2009). Nicotinamide phosphoribosyltransferase imparts human endothelial cells with extended replicative lifespan and enhanced angiogenic capacity in a high glucose environment. Aging cell, 8(2), 100–112. https://doi.org/10.1111/j.1474-9726.2009.00453.x
[10] Jadeja, R. N., Powell, F. L., Jones, M. A., Fuller, J., Joseph, E., Thounaojam, M. C., Bartoli, M., & Martin, P. M. (2018). Loss of NAMPT in aging retinal pigment epithelium reduces NAD+ availability and promotes cellular senescence. Aging, 10(6), 1306–1323. https://doi.org/10.18632/aging.101469
[11] Piedra-Quintero, Z. L., Wilson, Z., Nava, P., & Guerau-de-Arellano, M. (2020). CD38: An Immunomodulatory Molecule in Inflammation and Autoimmunity. Frontiers in immunology, 11, 597959. https://doi.org/10.3389/fimmu.2020.597959
[12] Amici, S. A., Young, N. A., Narvaez-Miranda, J., Jablonski, K. A., Arcos, J., Rosas, L., Papenfuss, T. L., Torrelles, J. B., Jarjour, W. N., & Guerau-de-Arellano, M. (2018). CD38 Is Robustly Induced in Human Macrophages and Monocytes in Inflammatory Conditions. Frontiers in immunology, 9, 1593. https://doi.org/10.3389/fimmu.2018.01593
[13] Aksoy, P., White, T. A., Thompson, M., & Chini, E. N. (2006). Regulation of intracellular levels of NAD: a novel role for CD38. Biochemical and biophysical research communications, 345(4), 1386–1392. https://doi.org/10.1016/j.bbrc.2006.05.042
[14] Camacho-Pereira, J., Tarragó, M. G., Chini, C., Nin, V., Escande, C., Warner, G. M., Puranik, A. S., Schoon, R. A., Reid, J. M., Galina, A., & Chini, E. N. (2016). CD38 Dictates Age-Related NAD Decline and Mitochondrial Dysfunction through an SIRT3-Dependent Mechanism. Cell metabolism, 23(6), 1127–1139. https://doi.org/10.1016/j.cmet.2016.05.006
[15] Barbosa, M. T., Soares, S. M., Novak, C. M., Sinclair, D., Levine, J. A., Aksoy, P., & Chini, E. N. (2007). The enzyme CD38 (a NAD glycohydrolase, EC 3.2.2.5) is necessary for the development of diet-induced obesity. FASEB journal: official publication of the Federation of American Societies for Experimental Biology, 21(13), 3629–3639. https://doi.org/10.1096/fj.07-8290com
[16] Escande, C., Nin, V., Price, N. L., Capellini, V., Gomes, A. P., Barbosa, M. T., O’Neil, L., White, T. A., Sinclair, D. A., & Chini, E. N. (2013). Flavonoid apigenin is an inhibitor of the NAD+ ase CD38: implications for cellular NAD+ metabolism, protein acetylation, and treatment of metabolic syndrome. Diabetes, 62(4), 1084–1093. https://doi.org/10.2337/db12-1139
[17] Chini, C., Tarragó, M. G., & Chini, E. N. (2017). NAD and the aging process: Role in life, death and everything in between. Molecular and cellular endocrinology, 455, 62–74. https://doi.org/10.1016/j.mce.2016.11.003
[18] Kellenberger, E., Kuhn, I., Schuber, F., & Muller-Steffner, H. (2011). Flavonoids as inhibitors of human CD38. Bioorganic & medicinal chemistry letters, 21(13), 3939–3942. https://doi.org/10.1016/j.bmcl.2011.05.022
[19] Tarragó, M. G., Chini, C., Kanamori, K. S., Warner, G. M., Caride, A., de Oliveira, G. C., Rud, M., Samani, A., Hein, K. Z., Huang, R., Jurk, D., Cho, D. S., Boslett, J. J., Miller, J. D., Zweier, J. L., Passos, J. F., Doles, J. D., Becherer, D. J., & Chini, E. N. (2018). A Potent and Specific CD38 Inhibitor Ameliorates Age-Related Metabolic Dysfunction by Reversing Tissue NAD+ Decline. Cell metabolism, 27(5), 1081–1095.e10. https://doi.org/10.1016/j.cmet.2018.03.016
[20] Scheibye-Knudsen, M., Mitchell, S. J., Fang, E. F., Iyama, T., Ward, T., Wang, J., Dunn, C. A., Singh, N., Veith, S., Hasan-Olive, M. M., Mangerich, A., Wilson, M. A., Mattson, M. P., Bergersen, L. H., Cogger, V. C., Warren, A., Le Couteur, D. G., Moaddel, R., Wilson, D. M., 3rd, Croteau, D. L., … Bohr, V. A. (2014). A high-fat diet and NAD(+) activate Sirt1 to rescue premature aging in cockayne syndrome. Cell metabolism, 20(5), 840–855. https://doi.org/10.1016/j.cmet.2014.10.005
[21] Wiley, C. D., Velarde, M. C., Lecot, P., Liu, S., Sarnoski, E. A., Freund, A., Shirakawa, K., Lim, H. W., Davis, S. S., Ramanathan, A., Gerencser, A. A., Verdin, E., & Campisi, J. (2016). Mitochondrial Dysfunction Induces Senescence with a Distinct Secretory Phenotype. Cell metabolism, 23(2), 303–314. https://doi.org/10.1016/j.cmet.2015.11.011
[22] Fang, E. F., Kassahun, H., Croteau, D. L., Scheibye-Knudsen, M., Marosi, K., Lu, H., Shamanna, R. A., Kalyanasundaram, S., Bollineni, R. C., Wilson, M. A., Iser, W. B., Wollman, B. N., Morevati, M., Li, J., Kerr, J. S., Lu, Q., Waltz, T. B., Tian, J., Sinclair, D. A., Mattson, M. P., … Bohr, V. A. (2016). NAD+ Replenishment Improves Lifespan and Healthspan in Ataxia Telangiectasia Models via Mitophagy and DNA Repair. Cell metabolism, 24(4), 566–581. https://doi.org/10.1016/j.cmet.2016.09.004
[23] Li, J., Bonkowski, M. S., Moniot, S., Zhang, D., Hubbard, B. P., Ling, A. J., Rajman, L. A., Qin, B., Lou, Z., Gorbunova, V., Aravind, L., Steegborn, C., & Sinclair, D. A. (2017). A conserved NAD+ binding pocket that regulates protein-protein interactions during aging. Science (New York, N.Y.), 355(6331), 1312–1317. https://doi.org/10.1126/science.aad8242
[24] Franceschi, C., & Campisi, J. (2014). Chronic inflammation (inflammaging) and its potential contribution to age-associated diseases. The journals of gerontology. Series A, Biological sciences and medical sciences, 69 Suppl 1, S4–S9. https://doi.org/10.1093/gerona/glu057