There has been considerable debate around the age-related decline of NAD+, particularly regarding whether or not it occurs in humans as it does in other species, such as mice. Today, as part of a two-part special, we are going to be taking a look at some of the evidence for the age-related decline of NAD+ in humans and some of the reasons why this likely happens.
What is NAD+?
NAD+ is a molecule that is present in all living cells and is essential for a myriad of cellular processes, including regulating metabolism, performing signaling, facilitating DNA repair and blood vessel growth, and regulating some aspects of aging.
In the body, NAD+ is created from simple building blocks, such as the amino acid tryptophan, and it is created in a more complex way via the intake of food containing nicotinic acid (NA), nicotinamide riboside (NR), nicotinamide mononucleotide (NMN), and other NAD+ precursors.
These different pathways ultimately feed into a salvage pathway, which recycles them back into the active NAD+ form. This salvage pathway, shown on the bottom right of the diagram, includes NAM, NMN, NAD+, and their associated steps.
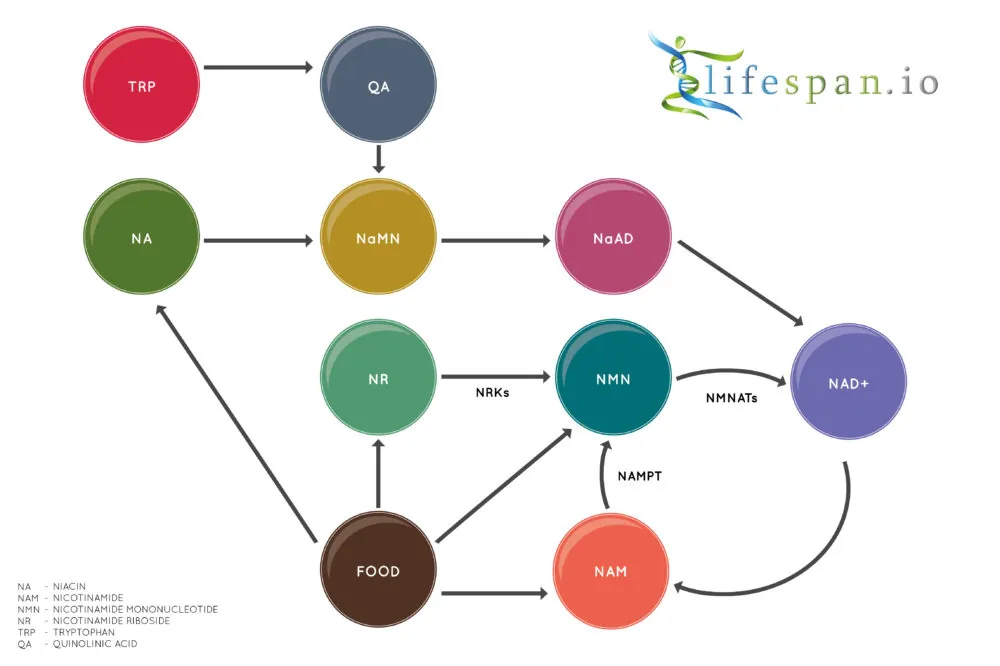
The evidence that NAD+ declines with age
A 2012 study reported that NAD+ levels decrease dramatically during aging in skin, and while the exact level of decline was not worked out, the average level of concentration seems to fall by a minimum of 50% during adult aging. The difference between newborns and adults, for example, appears to be several-fold lower, according to the study data [1].
For the brain, two studies used MRI to examine NAD+ and how its level changes during aging. The first study, conducted back in 2015, saw researchers measuring intracellular NAD+ and NADH concentrations and the NAD+/NADH ratio in the human brain, detecting the age-dependent changes in NAD contents and the redox state associated with aging [2].
The second study from 2019 also concluded that an age-dependent decline of the NAD+ levels in the brain was also observed [3]. Taken together, these two studies suggest that there is a decline of NAD+ in the brain of between ~10% to ~25% between the period of young adulthood and old age.
Another study has come along to muddy the waters and contradict the previous two [4]. In biology, nothing is ever simple, and according to this 12-person, placebo-controlled randomized trial, there was no appreciable difference in NAD+ levels in the brain between young and old trial participants.
The researchers of this new study suggest that their results show that NAD+ decline is not associated with chronological aging per se in human muscle or brain, though they do suggest that brain and muscle tissue can benefit from supplementation with nicotinamide riboside, a precursor of NAD+.
It should be noted that this study is currently in preprint on Biorxiv and has not as yet passed peer review. It is also worth noting that at least one of the authors (Charles Brenner) has a stake in patents and the company that owns and licenses the sale of nicotinamide riboside.
For the liver, a previous study showed that liver samples from people aged 60 had around 30% lower concentrations of NAD+ compared to people aged 45 [5].
Finally, the level of NAD+ present in the bloodstream has also been shown to decrease rapidly during aging, according to the results of a 2019 study [6]. This more recent study improved upon an older study [7], which suggested that there was only a small decrease in NAD+ levels; this was due to the newer study using a superior methodology compared to the older one, thus giving a more accurate view of what happens to NAD+ in the bloodstream during aging.
It should be noted that NAD+ levels in blood are typically many times lower than tissue levels, and it is still not clear how important NAD+ is in the blood are and what function or significance its presence has.
These findings are also supported by a wealth of animal studies and suggests that an age-related decline of NAD+ is common across a range of species, which includes mice and humans.
Some reasons why NAD+ declines with age
Based on both human and animal studies, it seems pretty clear that NAD+ levels do decline during aging in multiple tissues and in blood. However, the big question here is: why does NAD+ decline because of aging?
There are a number of mechanisms that have the potential of reducing NAD+ and could be part of this age-related decline.
Inflammaging and CD38
The most obvious and direct way in which NAD+ is reduced is via the activity of an NAD+ consuming enzyme linked to inflammation called CD38, which destroys NAD+, and its activity steadily rises during aging as systemic inflammation levels increase.
CD38 is a membrane-bound NADase that hydrolyzes NAD+ to nicotinamide and (cyclic-)ADP-ribose. It is associated with immune responses and energy metabolism, but it is also a NADase whose levels rise with aging, with a corresponding increase in NADase activity and a decrease of NAD+.
Therapies that reduce inflammation, particularly the age-related chronic systemic inflammation known as “inflammaging”, could reduce the presence of CD38, thus offering the potential to increase NAD+ [8].
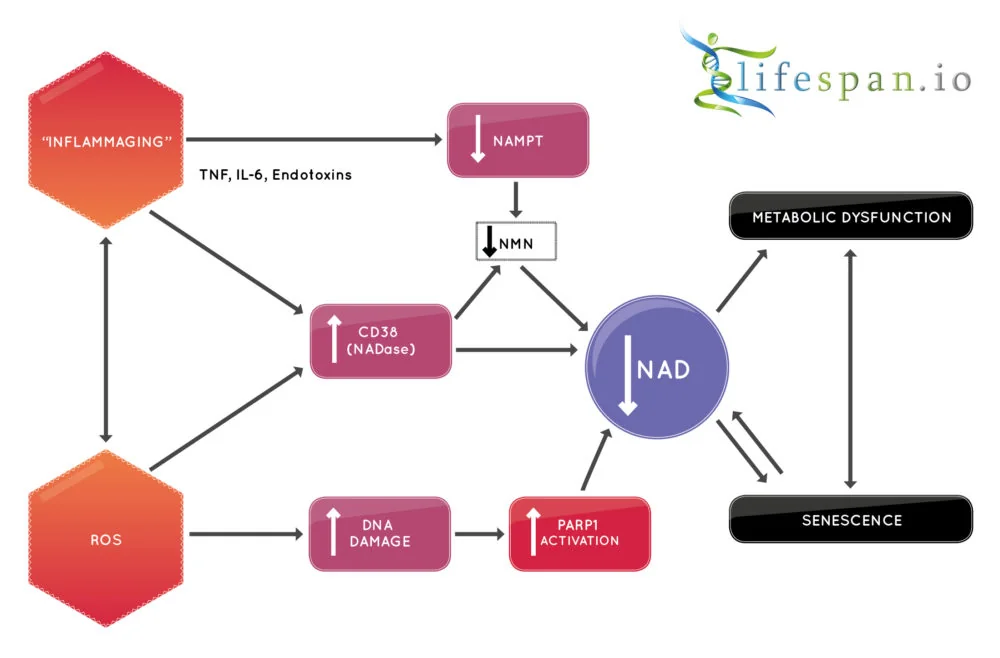
A new class of drugs known as senolytics, which purge the body of inflammatory senescent cells, is an example of a therapy that has the potential to reduce systemic inflammation and could improve NAD+ levels as a consequence.
It is worth noting at this point that it is still unclear whether or not NAD+ synthesis continues at the same rate in older individuals as younger ones, and while it seems clear that inflammaging and CD38 play a key role in consuming NAD+ as we age, there are also a number of other culprits that are involved.
DNA damage and PARPs
Poly-ADP-ribose polymerases (PARPs) are a group of related proteins involved in a number of cellular processes, such as DNA repair, genomic stability, and the programmed cell death known as apoptosis, which damaged cells undergo to remove themselves from the system.
PARP is a key driver of NAD+ catabolism, a set of metabolic processes that break down large molecules, including breaking down food molecules to provide energy and molecular components for anabolic reactions, using that released energy to repurpose the broken-down molecules for growth.
Specifically, PARP1 activity can be increased further in response to DNA damage and genotoxic stress, and given that DNA damage typically increases with age, that would mean more PARP activity and thus more NAD+ consumption [9]. In this way, PARP1 activity may serve as a regulator of NAD+ and mediate its age-related decline as the result of ever-increasing genomic instability that comes with age.
This suggests that therapies that can reduce PARP activity may be a viable approach here; certainly, in animal models of DNA damage, there is NAD+ depletion that can be reversed by inhibiting PARP [10-11]. Other approaches, such as NAD+ repletion via precursor supplementation, may also help to address excessive PARP consumption.
SARM1
The SARM1 protein is another way in which NAD+ levels may be depleted during aging. It was recently demonstrated that SARM1 is able to cleave NAD+, creating NAM, ADP ribose and cyclic ADP ribose [12-13]. Activation of SARM1 has been shown to reduce the available NAD+ pool, but when its activity is blocked, it reduces diabetic peripheral neuropathy and addresses axonopathy in animal models [14-16].
Axonopathy refers to a functional or structural defect in the axon or its terminal, and it is believed to be a precursor for the development and progression of various neurodegenerative diseases. For this reason, SARM1 has become a potential target for drug development; however, there is, at present, little data for SARM1 activity in neurons in the context of aging.
SARM1 is also expressed in other tissues, including the liver and kidneys, and its function in that context of age-related changes to its activity is as yet not fully understood, nor is how that function changes during aging. For this reason, it is still not clear if it plays a central role in age-related NAD+ decline.
Conclusion
This is the end of part one, and we will be delving further into the potential sources of NAD+ depletion in part two, which is coming soon.
Literature
[1] Massudi, H., Grant, R., Braidy, N., Guest, J., Farnsworth, B., & Guillemin, G. J. (2012). Age-associated changes in oxidative stress and NAD+ metabolism in human tissue. PloS one, 7(7), e42357.
[2] Zhu, X. H., Lu, M., Lee, B. Y., Ugurbil, K., & Chen, W. (2015). In vivo NAD assay reveals the intracellular NAD contents and redox state in healthy human brain and their age dependences. Proceedings of the National Academy of Sciences, 112(9), 2876-2881.
[3] Bagga, P., Hariharan, H., Wilson, N. E., Beer, J. C., Shinohara, R. T., Elliott, M. A., … & Detre, J. A. (2020). Single-Voxel 1H MR spectroscopy of cerebral nicotinamide adenine dinucleotide (NAD+) in humans at 7T using a 32-channel volume coil. Magnetic Resonance in Medicine, 83(3), 806-814.
[4] Elhassan, Y. S., Kluckova, K., Fletcher, R. S., Schmidt, M., Garten, A., Doig, C. L., … & Wilson, M. (2019). Nicotinamide riboside augments the human skeletal muscle NAD+ metabolome and induces transcriptomic and anti-inflammatory signatures in aged subjects: a placebo-controlled, randomized trial. BioRxiv, 680462.
[5] Zhou, C. C., Yang, X., Hua, X., Liu, J., Fan, M. B., Li, G. Q., … & Wang, P. (2016). Hepatic NAD+ deficiency as a therapeutic target for non-alcoholic fatty liver disease in ageing. British journal of pharmacology, 173(15), 2352-2368.
[6] Clement, J., Wong, M., Poljak, A., Sachdev, P., & Braidy, N. (2019). The plasma NAD+ metabolome is dysregulated in “normal” aging. Rejuvenation research, 22(2), 121-130.
[7] Guest, J., Grant, R., Mori, T. A., & Croft, K. D. (2014). Changes in oxidative damage, inflammation and [NAD (H)] with age in cerebrospinal fluid. PLoS One, 9(1), e85335.
[8] Tarragó, M. G., Chini, C. C., Kanamori, K. S., Warner, G. M., Caride, A., de Oliveira, G. C., … & Jurk, D. (2018). A potent and specific CD38 inhibitor ameliorates age-related metabolic dysfunction by reversing tissue NAD+ decline. Cell metabolism, 27(5), 1081-1095.
[9] Wang, G., Han, T., Nijhawan, D., Theodoropoulos, P., Naidoo, J., Yadavalli, S., … & McKnight, S. L. (2014). P7C3 neuroprotective chemicals function by activating the rate-limiting enzyme in NAD salvage. Cell, 158(6), 1324-1334.
[10] Fang, E. F., Scheibye-Knudsen, M., Brace, L. E., Kassahun, H., SenGupta, T., Nilsen, H., … & Bohr, V. A. (2014). Defective mitophagy in XPA via PARP-1 hyperactivation and NAD+/SIRT1 reduction. Cell, 157(4), 882-896.
[11] Scheibye-Knudsen, M., Mitchell, S. J., Fang, E. F., Iyama, T., Ward, T., Wang, J., … & Mangerich, A. (2014). A high-fat diet and NAD+ activate Sirt1 to rescue premature aging in cockayne syndrome. Cell metabolism, 20(5), 840-855.
[12] Summers, D. W., Gibson, D. A., DiAntonio, A., & Milbrandt, J. (2016). SARM1-specific motifs in the TIR domain enable NAD+ loss and regulate injury-induced SARM1 activation. Proceedings of the National Academy of Sciences, 113(41), E6271-E6280.
[13] Essuman, K., Summers, D. W., Sasaki, Y., Mao, X., DiAntonio, A., & Milbrandt, J. (2017). The SARM1 Toll/interleukin-1 receptor domain possesses intrinsic NAD+ cleavage activity that promotes pathological axonal degeneration. Neuron, 93(6), 1334-1343.
[14] Cheng, Y., Liu, J., Luan, Y., Liu, Z., Lai, H., Zhong, W., … & Huang, R. (2019). Sarm1 gene deficiency attenuates diabetic peripheral neuropathy in mice. Diabetes, 68(11), 2120-2130.
[15] Gilley, J., Ribchester, R. R., & Coleman, M. P. (2017). Sarm1 deletion, but not WldS, confers lifelong rescue in a mouse model of severe axonopathy. Cell reports, 21(1), 10-16.
[16] Gerdts, J., Brace, E. J., Sasaki, Y., DiAntonio, A., & Milbrandt, J. (2015). SARM1 activation triggers axon degeneration locally via NAD+ destruction. Science, 348(6233), 453-457.