In a preprint published in bioRxiv, researchers have published their findings in applying an epigenetic clock to the axolotl, a salamander species that does not age like humans.
More than just regeneration
Axolotls, and salamanders more generally, are well-known for their regenerative capabilities, being able to grow back lost limbs [1]. These amphibians, similarly to naked mole rats, have not been found to age the way we do: they do not decline in physical function, their regeneration continues throughout life, they live a very long time, and their lifespan curve does not look like other species’ [2]. Despite their regenerative power, they are also notably resistant to cancer, even when directly injected with carcinogens [3].
In fact, with an average lifespan of 10-13 years, which is very high for a small amphibian, the axolotl does not live as long as other salamanders. This has made it attractive as a research target, as modern transfection and genetic analysis techniques that are normally associated with murine research have been developed to work with this species [4].
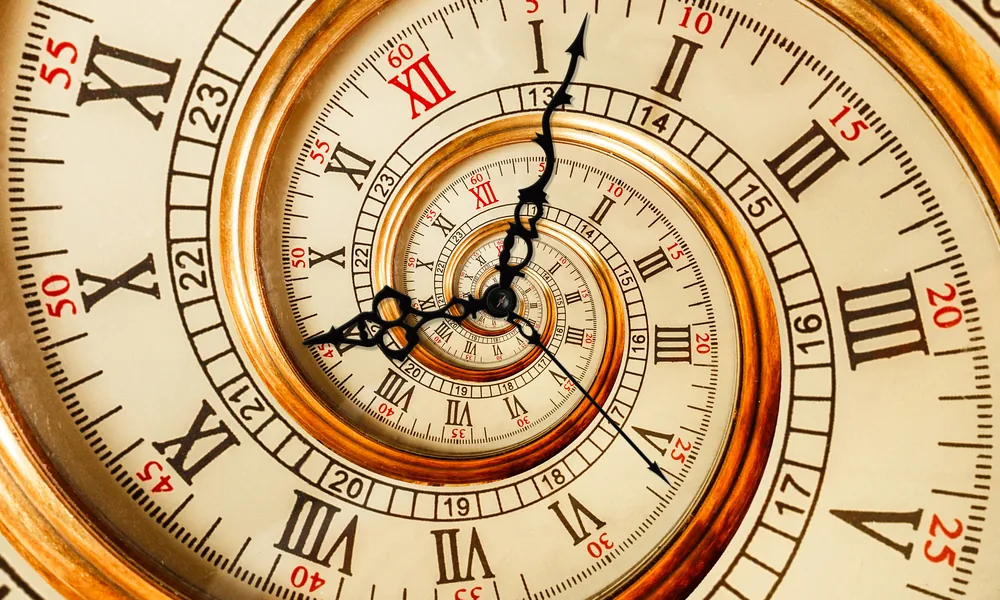
Read More
While there are known pan-mammalian epigenetic clocks that work on people, mice, and naked mole rats [5], the axolotl is an amphibian, not a mammal. However, as similarities have been found between the African clawed frog (another amphibian) and mammals [6], the researchers surmised that epigenetic aging is similar enough between these species for this line of inquiry to be effective. However, unlike mammals, amphibians’ epigenetic ages do not vary considerably between tissues.
No differences between old and young
The researchers found 5,386 epigenetic CpG sites that axolotls, clawed frogs, and mammals have in common. They then attempted to use an algorithm to create a clock with these sites that works over the axolotl’s lifespan. This effort failed completely: there appeared to be no significant correlation between epigenetic age and chronological age, whether they attempted to build a single-tissue or pan-tissue clock. This was in spite of having more than a sufficient number of high-quality samples to use, and an even closer examination failed to reveal any significant differences between the methylation of a 3.55-year-old axolotl and a 9.83-year-old axolotl.
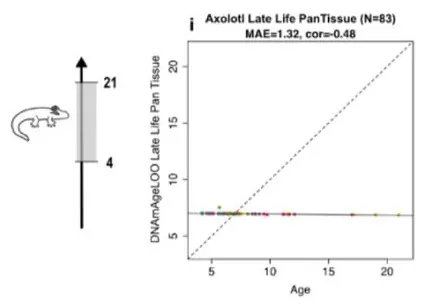
However, the axolotls did appear to be aging earlier in life, so the researchers developed a clock that was only trained on animals up to 4 years of age. This clock was able to predict ages of these salamanders within a few months. The researchers, therefore, concluded that axolotl epigenetic aging is “biphasic”: they age up to a certain point, and then they simply stop epigenetically aging to any measurable degree.
An axolotl early-life clock was also demonstrated to be compatible with epigenetic clocks for clawed frogs and people. According to this dual-species clock, very young humans had similar methylation as very young axolotls, but as the humans aged, the axolotls did not age with them.
A closer examination revealed the similarities and differences. HOX genes, which are associated with organismal development, all change methylation in the early lives of people, frogs, and axolotls, demonstrating growth. However, CpGs that were associated with an increase in mortality risk, such as cancer, simply did not exist in the axolotl clock.
Even limb regeneration did not appear to age these animals. Cutting off an axolotl’s tail six times in a row did not have any significant effects on that area’s epigenetic age, although there was a trend towards being marked as epigenetically older. On the other hand, amputating one of its limbs three times made the epigenetics of that repeatedly regrown limb younger. The researchers found that this correlated with the expression of factors that are known to affect cells at various stages of differentation, and they posit that this “dynamic regulation may be central to the epigenetic rejuvenation that takes place upon limb regeneration.”
Medical professionals, and people who have suffered severe injuries, have desired to harness the salamander’s regeneration for a long time. Now, it appears that this regeneration might also come along with significant anti-aging effects. Applying these effects to mammals, however, will require significant amounts of time and experimentation.
Literature
[1] Brockes, J. P., & Kumar, A. (2008). Comparative aspects of animal regeneration. Annual review of cell and developmental biology, 24(1), 525-549.
[2] Yun, M. H. (2021). Salamander insights into ageing and rejuvenation. Frontiers in cell and developmental biology, 9, 689062.
[3] Ingram, A. J. (1971). The reactions to carcinogens in the axolotl (Ambystoma mexicanum) in relation to the ‘regeneration field control’hypothesis. Development, 26(3), 425-441.
[4] Murawala, P., Oliveira, C. R., Okulski, H., Yun, M. H., & Tanaka, E. M. (2022). Baculovirus Production and Infection in Axolotls. In Salamanders: Methods and Protocols (pp. 369-387). New York, NY: Springer US.
[5] Lu, A. T., Fei, Z., Haghani, A., Robeck, T. R., Zoller, J. A., Li, C. Z., … & Singh, K. (2023). Universal DNA methylation age across mammalian tissues. Nature aging, 3(9), 1144-1166.
[6] Zoller, J. A., Parasyraki, E., Lu, A. T., Haghani, A., Niehrs, C., & Horvath, S. (2024). DNA methylation clocks for clawed frogs reveal evolutionary conservation of epigenetic aging. GeroScience, 46(1), 945-960.