In Stem Cell Reports, researchers have described how cell subpopulations and division affect the results of epigenetic clocks.
Good, but never perfect
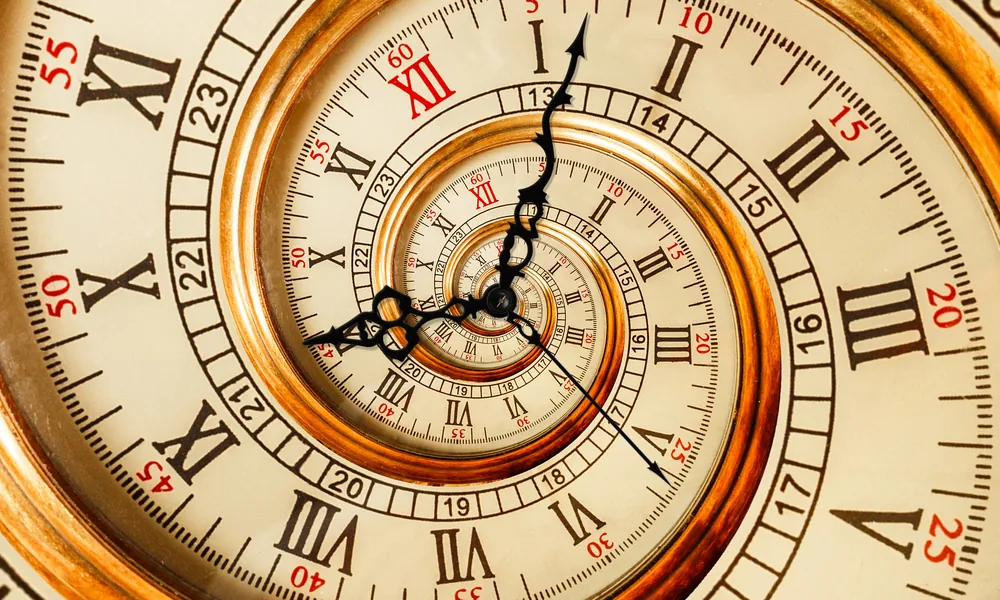
Read More
As these researchers note, epigenetic clocks have had successes in analyzing the impact of things that impact biological age, such as the effects of stress and stress reduction [1] and of progeria [2]. However, such things as differentiation of stem cells are known to change the epigenetic markers used by these clocks [3], raising questions about their reliability.
One way of avoiding this is to use single-cell clocks, but those lack the overall precision of other clocks [4] and have not yet been developed for every cell type. Additionally, some tissues have been found to age more slowly than others [5]. Therefore, these researchers dived more deeply into this subject, attempting to find out how clocks are affected by differentiation, tissue type, and cellular proliferation.
Even similar cells age at different rates
The researchers used three types of clocks to analyze tissues in this study: tissue-specific, pan-tissue for a single species, and two universal clocks that measure age for any mammalian species.
Their first experiment was on muscle tissue derived from mice. The stem cells of muscle tissue were calculated as being less than half of the epigenetic age of differentiated muscle cells, even using a sample taken from a single mouse. The universal clocks also found that stem cells were epigenetically younger, although this effect was far less striking.
Even the type of stem cell has an effect. In bone marrow, hematopoietic stem and progenitor cells (HSPCs), which can differentiate towards multiple fates, were examined alongside progenitors committed to lymph or blood cell (myeloid) fates along with differentiated cells. Curiously, HSPCs trended towards being the most aged of this group, while the lymphoid group trended towards being the least. This difference reached statistical significance in the universal clocks, with lymphoid-fated stem cells being reported as the epigenetically youngest with the other three clustered together.
While previous work has found differences in human intestinal tissue between stem cell-rich crypts and differentiated cell-rich villi [6], these researchers found no difference in their sample. They suggest that this difference may be species-specific or due to sampling issues.
Then, the researchers turned to epithelial tissues: specifically, the esophagus, tongue, skin, and lungs. Skin and tongue cells had largely similar measurements between stem cells and differentiated cells, while esophageal stem cells trended towards being slightly older. However, in lung tissue, the pan-tissue clock reported that differentiated cells were twice as old as the stem cells, and the universal clocks reported ages in the decades, far longer than how long any mouse has ever actually lived.
Dividing cells age much faster
With these results in hand, the researchers then looked at cellular division. Epithelial issues that had more cellular divisions were epigenetically older than cells with fewer divisions. Repeatedly dividing stem cells from the antral gland in the stomach, the researchers found that cells that had divided only once were younger than cells that had divided 10 times and far younger than cells that had divided 19 times, according to both pan-tissue and universal clocks. Similar results were found with stem cells that had been allowed to repeatedly divide.
The researchers then went back to whole tissue and found that these results also applied there. In muscle tissue, cell types that do not divide often (quiescent cells) remained relatively young in older animals, while precursor cells that divide frequently were much older. This was compounded by the fact that the number of such rapidly dividing cells declines with age. In muscle tissue, these cells are rare enough that they are unlikely to affect a whole-tissue clock; however, their effects on blood tissue, despite efforts to account for this fact [7], remain “experimentally unverified” according to these researchers.
These results have led the researchers to hypothesize that accumulated DNA damage, and not just epigenetic alterations, leads to an increase in epigenetic age according to clocks. As previous research has found that at least some of this damage can be repaired [8], this offers an important insight for people developing epigenetic interventions.
Literature
[1] Poganik, J. R., Zhang, B., Baht, G. S., Tyshkovskiy, A., Deik, A., Kerepesi, C., … & Gladyshev, V. N. (2023). Biological age is increased by stress and restored upon recovery. Cell Metabolism, 35(5), 807-820.
[2] Horvath, S., Oshima, J., Martin, G. M., Lu, A. T., Quach, A., Cohen, H., … & Raj, K. (2018). Epigenetic clock for skin and blood cells applied to Hutchinson Gilford Progeria Syndrome and ex vivo studies. Aging (Albany NY), 10(7), 1758.
[3] Bock, C., Beerman, I., Lien, W. H., Smith, Z. D., Gu, H., Boyle, P., … & Meissner, A. (2012). DNA methylation dynamics during in vivo differentiation of blood and skin stem cells. Molecular cell, 47(4), 633-647.
[4] Hernando-Herraez, I., Evano, B., Stubbs, T., Commere, P. H., Jan Bonder, M., Clark, S., … & Reik, W. (2019). Ageing affects DNA methylation drift and transcriptional cell-to-cell variability in mouse muscle stem cells. Nature communications, 10(1), 4361.
[5] Horvath, S., Mah, V., Lu, A. T., Woo, J. S., Choi, O. W., Jasinska, A. J., … & Coles, L. S. (2015). The cerebellum ages slowly according to the epigenetic clock. Aging (Albany NY), 7(5), 294.
[6] Lewis, S. K., Nachun, D., Martin, M. G., Horvath, S., Coppola, G., & Jones, D. L. (2020). DNA methylation analysis validates organoids as a viable model for studying human intestinal aging. Cellular and molecular gastroenterology and hepatology, 9(3), 527-541.
[7] Zhang, Z., Reynolds, S. R., Stolrow, H. G., Chen, J. Q., Christensen, B. C., & Salas, L. A. (2024). Deciphering the role of immune cell composition in epigenetic age acceleration: Insights from cell‐type deconvolution applied to human blood epigenetic clocks. Aging Cell, 23(3), e14071.
[8] Beerman, I., Seita, J., Inlay, M. A., Weissman, I. L., & Rossi, D. J. (2014). Quiescent hematopoietic stem cells accumulate DNA damage during aging that is repaired upon entry into cell cycle. Cell stem cell, 15(1), 37-50.